Energy Impact Partners: A primer on the next three decades of the energy transition - Part 4
Energy Impact Partners research lead, Andy Lubershane, releases a five-part series examining technologies needed to fully decarbonize the energy system, relying on as few technology “moonshots” as possible.
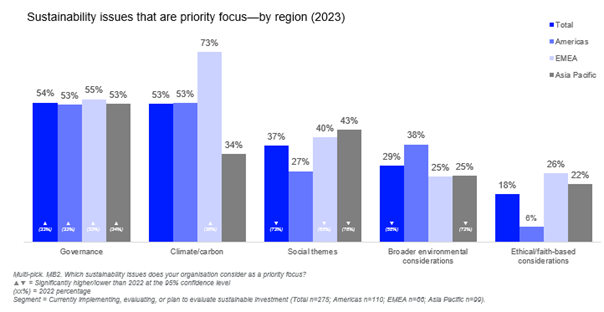
This article is the fourth of a five-part series examining the technology we’ll need to fully decarbonize our energy system, relying on as few technology “moonshots” as possible.
I. The Electric Future We Need
II. Two Storage Technology Revolutions
III. Cheaper, Deeper Offshore Wind: completing our renewable resource portfolio
IV. Clean Heat
Wind and solar power are essentially substitutes for heat — for example, the heat we produce by burning coal, which we use to boil water to produce steam, which spins a turbine, which in turn rotates a generator to create electricity. Or, the heat we produce by burning oil in an engine, in order to pump a piston, to rotate some gears which activate the drivetrain of a vehicle.
In each of the processes outlined above, heat is an intermediate product. We’re not after heat for its own sake. Hence, renewable energy substitutes can completely circumvent the need for heat by taking completely different steps to accomplish the same outcome.
Sometimes, though, we still want heat for its own sake. Sometimes we just want something to be hotter. In fact, for the vast majority of human history, most of the non-food energy sources we collected were used to warm our buildings, cook our food, or melt metals.
For many of these basic processes, we have yet to find an ideal substitute for burning fossil fuel; and unfortunately, electricity on its own doesn’t seem like it will do the trick. So, if we’re going to transition these activities to clean energy — i.e. to a value chain that begins with renewable power generation — we’re going to need alternatives.
Our heating needs fall into two broad categories, which will probably require different types of solutions:
1. Space heating for buildings: In cold weather, conditioning the spaces we live in to a comfortable temperature requires so-called “low-grade heat”, typically in the 70–100 degree Fahrenheit range.
2. Process heating for manufacturing: Many industrial processes rely on “high grade heat” — which ranges from hundreds of degrees to thousands of degrees Fahrenheit — usually in order to transform a material in some form or fashion.
Space heating: the promise and shortcomings of electric heat pumps
Let’s start with buildings. Today, about 10% of energy consumed in the US is used to heat our homes, offices, warehouses, hospitals, factories, and board game stores.
Source: EIA Annual Energy Outlook 2020; Bloomberg New Energy Finance, “Decarbonizing US Heat: Getting Started”, June 2019
A significant plurality of that heat comes from burning natural gas in a central furnace or boiler. A small minority of buildings — mostly in the Northeast — burn oil instead of gas. But a growing share of buildings — mostly in the warmer-climate Southern states — rely on electric heat pumps.
Heat pumps are a well-established technology that employ electricity and a refrigerant to gather ambient heat from one space, and transfer it into another. Every household refrigerator has a heat pump, which gathers heat from inside of the refrigerator and jettisons it out. The ‘magic’ of this technology is that it can keep on gathering heat from a fairly cold space — e.g. the 35 degree Fahrenheit space inside of your refrigerator — and pumping that heat into a space that’s already much warmer — e.g. your comfortable, 70 degree kitchen.
Even more magically, heat pumps are able to perform this feat with greater than 100% efficiency. What that means is they can deliver more energy, in the form of hot air, than the energy they take to run, in the form of electricity. This makes heat pumps much more efficient than what’s called “resistance heaters” — which are kind of like old-fashioned incandescent light bulbs. (Remember how hot those would get?) At best, resistance heating can approach 100% efficiency, while heat pumps can easily achieve over 200% efficiency, on average, even in fairly cold climates like the Northern US.
Heat pumps come with one additional benefit: they can be made reversible, which means the same unit can serve as both a heater in the winter, and an air conditioner in the summer. This ability improves their cost-effectiveness tremendously for new buildings, or for older buildings without existing air conditioning systems.
They sound like a wonder-technology, and they are. They’re certain to play a significant role in the decarbonization of space heating for buildings. Because of cost reductions, market acceptance, and a temporary spike in the price of natural gas in the early aughts, nearly half of new homes built in the US are now built with heat pumps.
Source: US Census Bureau, Characteristics of New Housing
However, kind of like Lithium-ion batteries for energy storage, heat pumps are not quite wonderful enough to be the only solution we’ll ever need.
Electric heat pumps face three primary challenges:
1. The very cold weather challenge: Heat pumps’ magical efficiency does not hold up in very cold weather. Below freezing, their efficiency drops precipitously, and they become, essentially, more expensive resistance heaters. In order to operate at all below about 5 degrees Fahrenheit, they need specialized cold weather designs. This isn’t necessarily a deal-breaker in most of the US, but it compounds the next challenge…
2. The winter electric delivery challenge: Converting heating systems to electric heat pumps will, of course, increase the need for clean, renewable electricity. That’s generally a good thing. But it will also put an enormous strain on the electric transmission & distribution grid — and that’s on top of the strain that planners are already anticipating from the rise of electric vehicles. In cold climate zones, where heat pumps will be operating least efficiently, a complete conversion to electric heating could increase the peak demand for electricity, on the coldest winter nights, by more than three-fold. Making matters worse, electric vehicles are also less efficient in cold weather — about 25–30% less efficient below freezing than in the mid-70s — so they’ll also need to draw more energy from the grid in the winter. For illustrative purposes, the chart below presents a model of electricity demand for my home state of Maine, given complete electrification of both transport and heat. (Orange represents space heating demand, which causes aggregate demand to surge in the winter.)
Source: “A New Energy Direction for Maine: Pathway to a Zero Carbon Economy by 2050”, Rich Silkman, Jan 2020
3. The cost-effectiveness challenge: Heat pumps have already proven to be the most cost-effective option for new homes in relatively warm climate zones — e.g. the US Southeast. But even in those regions they’re still not competitive enough to displace existing natural gas based heating systems; and they remain a premium option for new homes in cold climate zones.
Source: “Analysis of Residential Heat Pump Potential and Economics”, NYSERDA, Jan 2019
None of these challenges is, in itself, a deal-breaker for the prospect of a full-scale conversion to electric heating, but all told they make the cost of such a conversion daunting. Hence, I believe this is another area where the energy transition will not be satisfied by technological incrementalism. Specifically, in the decades to come, we need to see:
1. Cheaper, no-backup necessary electric heat pumps. Super-cold-weather-capable heat pumps are already available today from major manufacturers, and the existing technology is generally considered to be ‘good enough’. But there’s still room for improvement in terms of cost, reliability, and efficiency. One option is ground-source heat pumps, which solve the efficiency problem by burrowing down to capture heat from below the frost line, underground.
2. Efficiency gains for heat distribution in older buildings. Energy isn’t only lost during the production of heat; it’s also lost as heat is distributed around a building. In particular, we need to improve the distribution of heat in older buildings that move it around in hot water or steam.
3. A dramatic reduction in the amount of heat that each building requires. In addition to the age-old technology best known as “putting on a sweater”, this category could also include innovative approaches to building ventilation and air quality; better insulation; and other improvements to the building ‘envelope’.
4. Efficient hydrogen-fueled furnaces and heat pumps. The first article in this series laid out some of the pros and cons of hydrogen as a major global ‘energy medium’. As that article explained, space heating is one of the energy end uses that could benefit the most from cheaper production of clean hydrogen. Burning hydrogen as a primary heating fuel (or heat pump fuel) solves the first two challenges for electric heat pumps: hydrogen is indifferent to very cold temperatures; and it can be distributed via existing gas infrastructure, rather than electrical wires. If hydrogen heating systems can be manufactured cheaply enough, alongside hydrogen itself, then they could end up playing a major role in the decarbonization of heat.
Industrial process heat: the next frontier
It turns out that making things super, super hot is one of the hardest things to do, cost-effectively, with clean energy. At the temperatures required by many industrial processes, most electric heating systems would simply melt. Heat pumps are magical, but not magical enough to pump heat into a 1000 degree furnace.
Unfortunately, industrial heat is one of the biggest consumers of energy in the world. In the US, we consume more energy to heat manufacturing processes than we consume to heat all of our buildings combined. Today, only about 10% of manufacturing process heat is electrified; the rest is produced by burning fossil fuels. Manufacturers report that they have already done just about all of the cost-effective ‘fuel switching’ that they know how to do. A National Renewable Energy Lab study of society-wide electrification opportunities came to the same conclusion.
Industry also consumes fossil fuels as a feedstock for specific processes and outputs — for example, making plastics. Complicating matters, some of these processes aren’t easy to separate into ‘heating’ and ‘feedstock’; the two are sometimes tightly bound together into a single chain of combustion and chemical reactions. For example, in order to make steel, we can’t simply substitute one heating source for another; we use a specific form of coal called “coke” which acts as both a heat source and a chemical reducing agent.
Hence, decarbonizing industrial heat is one of the most difficult challenges we’ll need to solve in the coming decades. It’s an especially tough set of challenges for private sector investment to tackle, as it’s defined by enormous capital requirements; risk-averse industrial buyers operating on thin margins; and a hyper-fragmented landscape of specialized processes that each require distinctive solutions. I’ve yet to find a technology category better-suited to government-backed R&D and commercialization. There’s no sector more in need of a carbon price…
In sum: Out of all the tech categories I’m highlighting in this series, industrial process heat has basically zero chance of moving fast enough without policy intervention.
I’m sorry to end this section on a less-than-hopeful tone, but that’s the reality of the situation. Fortunately, there’s more hope in the next (and final) article in this series, which focuses on technology we need to keep the electrical grid up and running through this era of dramatic change.